ABOUT OUR RESEARCH
The UWS Nuclear Physics Research Group has been established for almost 30 years. Our research has traditionally been focussed on the study of the structure and properties of exotic atomic nuclei using gamma-ray and charged-particle spectroscopy. Our research is typically conducted at overseas nuclear-physics laboratories such as JYFL in Finland, Argonne National Laboratory in the USA, ISOLDE at CERN, and TRIUMF in Vancouver. We are leading programmes in a number of different areas of nuclear physics at these laboratories. We are members of SUPA, the Scottish Universities Physics Alliance, within which we are leading nuclear physics experiments at the new SCAPA facility – the Scottish Centre for the Application of Plasma-based accelerators. Our research has traditionally been fundamental in nature, studying the structure and properties of atomic nuclei, but we are developing themes of research in areas of applied nuclear physics, with the intention of developing impact to society, industry and the economy. Our research has been continuously funded by research councils (EPSRC and STFC) for almost 30 years. We are presently funded by an STFC Consolidated Grant and an STFC Project Grant (AGATA).
RESEARCH AREAS
-
Octupole correlations in nuclei
-
The nuclear dipole response
-
Study of neutrin-rich nuclei using binary grazing reactions
-
High resolution beta-decay stiudies
-
The measurement of lifetimes of nuclear states
-
Neutron-proton interactions
-
Exoic proton-rich nuclei above Sn100
-
Nuclear astrophysics
-
AGATA - the Advanced Gamma Tracking Spectrometer
-
Research with SCAPA
-
Detector development
PROJECT DESCRIPTIONS
Octupole correlations in nuclei
One of our areas of research is the study of nuclei with strong octupole correlations which leads to the nucleus having a reflection asymmetric shape, often said to be a “pear shape”. The atomic nucleus is often considered to be a spherical ball of strongly interacting neutrons and protons, but this is not always the case. Nuclei can take on different shapes depending on the different interactions between the constituent nucleons. The first deformed nuclei that were identified were prolate, shaped like a rugby ball. These nuclei were identified by their large electric quadrupole moments, and their tendency for form collective rotational states - a mode of excitation that is quantum mechanically not possible for a spherical nucleus. Some nuclei were also identified to be oblate, shaped like a pumpkin or a squashed sphere. A more novel shape is that of a “pear shape” in which the nucleus takes on a reflection-asymmetric shape with axial symmetry but with one end being more pointed than the other end like a pear. Suggestions of this shape first came from alpha-decay experiments in the 1950s where low-lying negative-parity states were explained by the existence of octupole correlations. Since then, there have been a number of theoretical and experimental studies of octupole-deformed shapes. There are a number of spectroscopic characteristics which are associated with strong octupole correlations in nuclei. The pear-shaped nucleus gives rise to a displacement of the centre of mass and the centre of charge in the nucleus which leads to a large intrinsic electric dipole (E1) moment. This is apparent experimentally in the form of strong electric-dipole transitions between positive- and negative-parity states. In many octupole deformed nuclei, rotational like bands of negative-parity states are often built on the low, lying negative parity states. Octupole-deformed shapes are therefore characterized by rotational-like bands of positive and negative parity states connected by strong E1 transitions. The octupole-deformed shape will also have a large electric-octupole (E3) moment which can be measured in certain types of experiments. The strongest octupole corelations, and therefore the most pronounced reflection-asymmetric shapes in nuclei, are found in four regions of the nuclei chart. These regions are related to the so-called octupole magic numbers which correspond to regions where pairs of orbitals with the correct properties come close enough together to interact. These nucleon numbers are 134, 90, 56, and 34. The main regions of octupole correlations are nuclei near 224Th (Z=90, N=134), near 146Ba (Z=56, N=90) and near 112Ba (Z=56, N=56). We have ongoing research programmes studying nuclei in these regions. For example, we have recently led experiments studying the excited states of the nuclei 222Th and 224U at Argonne National Laboratory and at the JYFL Laboratory. We are also leading a programme of Coulomb-excitation measurements with radioactive-ion beams studying Ra (Z=88), Rn (Z=86) and Ba (Z=56) nuclei at the REX-ISOLDE facility with Miniball. We also have an ongoing programme studying the very neutron-deficient nuclei in the A=120 region, which includes the study of octupole correlations heading towards 112Ba (Z=56, N=56). We are planning to continue this theme of research with experiments at JYFL and at Argonne, and also at future facilities such as FRIB and SPES.
The Galileo gamma-ray spectrometer which was used in our experiment to study radium-218
The study of neutron-rich nuclei using binary grazing reactions
Binary grazing and deep-inelastic reactions have been used extensively during the last few decades in studies of the structure of neutron-rich nuclei over a wide range of nuclear masses. The coupling of large solid-angle magnetic spectrometers for the detection of ejectiles to large arrays of Ge γ-ray detectors has represented a very significant experimental advance over earlier experiments with no ejectile identification. Recent work at the INFN Legnaro National Laboratory has used binary grazing reactions initiated by a beam of 235-MeV 36S ions incident on a 208Pb target to study neutron-rich nuclei in the sdpf shell. The PRISMA magnetic spectrometer was used to detect and identify projectile-like ions and coincident gamma rays were detected by Ge detector arrays; the CLARA array of escape-suppressed Ge detectors was used in a spectroscopy experiment whereas the AGATA γ-ray tracking array was employed in lifetime measurements with the recoil distance method. Published works from the experiments have involved studies of the neutron-rich isotopes 33Si, 36Si, 34P, 35P, 36P, 37P, 38P, 37S, 39S, 40S, 41S, and 38Cl. The studies were mainly concerned with the role of negative-parity intruder orbitals in the structure of neutron-rich nuclei on the periphery of the island of inversion, which is centred on 32Mg, and on the description of such nuclei using state-of-the-art shell-model calculations.
The PRISMA and CLARA spectrometers at the INFN Legnaro National Laboratory
High resolution beta-decay studies with GRIFFIN
The Gamma Ray Infrastructure for Fundamental Investigations of Nuclei (GRIFFIN) spectrometer is located in the low-energy ISAC-I experimental hall at the TRIUMF facility in Vancouver, Canada. GRIFFIN incorporates 16 Compton-suppressed high-purity germanium Clover gamma-ray detectors with an efficiency of around 10% at 1 MeV. Several ancillary detector systems can be used with GRIFFIN including PACES (Pentagonal Array for Conversion Electron Spectroscopy) and up to 8 lanthanum-bromide [LaBr3(Ce)] detectors. All detector systems are coupled to a triggerless, high-throughput digital data acquisition system. GRIFFIN is uniquely placed to exploit the high-intensity radioactive ion beams provided by the TRIUMF-ISAC facility. The ability to detect very weak gamma-ray transitions at high resolution makes it particularly useful in elucidating the structure of nuclei populated in beta decay. High-energy transitions approaching the beta-decay Q-value may be observed. Both gamma and beta-decay branching ratios can be measured with high precision, providing constraints (for example) on the angular momentum of excited nuclear states. GRIFFIN is ideal for studying ‘superallowed’ (Fermi) beta decays through measurements of ground state to ground state 0+0+ beta transitions, offering insight regarding the Cabibo-Kobayashi-Maskawa (CKM) matrix and the coupling amplitudes between different quark flavours. Members of the UWS Nuclear Physics Research group are leading efforts to explore the structure of heavy actinide nuclei with GRIFFIN, including radium (Z=88) and thorium (Z=90) isotopes, many of which are proposed to exhibit highly collective, reflection-asymmetric octupole shapes near their ground state. The intensity of actinide beams available at the TRIUMF-ISAC facility are among the highest globally. GRIFFIN may be used together with PACES to recover the intensity of highly-converted nuclear de-excitations. Combined with information regarding the lifetimes of nuclear-excited states from the LaBr3(Ce) detectors, a comprehensive picture of the nucleus emerges.
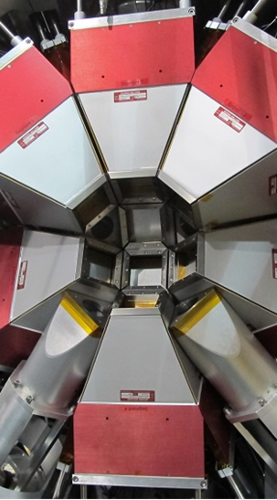
Photo of the east hemisphere of GRIFFIN with BGO Compton-suppression shields installed around the HPGe clovers. Compton-suppressed LaBr3(Ce) detectors are visible to either side of the bottom clover.
The nuclear dipole response
The nuclear dipole response has an electric and a magnetic part. Both contributors exhibit a variety of features with a multitude of implications, such as the violation of fundamental symmetries, extreme astrophysical objects such as neutron stars, the synthesis of heavy elements, or the dynamics of supernova type-II explosions.
The electric dipole response (E1R) can be classified in low-lying quadrupole-octupole coupled excitations, which are particular interesting if the nucleus has quadrupole but also (so far established in less than a handful of cases) octupole correlations in its ground state. The E1R is dominated by the Giant Dipole Resonance (GDR) at comparably high excitation energy beyond the particle thresholds. On the GDR’s low-energy tail near the proton and neutron evaporation threshold a less strong additional enhancement of E1 strength is found. This, so called Pygmy Dipole Resonance (PDR) is often visualized as an out-of-phase motion of a neutron skin formed by excess neutrons and an isospin saturated core composed of an almost equal number of protons and neutrons. This mode provides an additional wide-open doorway in particle-capture reactions in astrophysical scenarios as the slow and rapid neutron capture processes. Furthermore, the skin picture of ‘pure’ neutron matter suggests connections via the symmetry energy, which enters the nuclear Equation-of-State, to neutron stars.
The magnetic dipole response splits into the scissors resonance near 3 MeV and the Gamow-Teller Resonances near the particle threshold. The latter has strong implications for beta decay probabilities and even more so neutrino-nucleus scattering cross sections. The latter are deemed to be the mechanism driving the explosion of type-II Supernova.
Our group has become interested to benchmark these excitations in nuclei with equal proton and neutron number. For example, in the case of the PDR, this allows to disentangle shell effects (in our nuclei) from neutron-skin+shell effects in more neutron rich nuclei. We have an ongoing experimental program for the lightest stable Ni isotopes 58 and 60 and aim to investigate 24-Mg and 28-Si. As experimental tools we use the scattering of real photons, bremsstrahlung for the excitation strength and fully-polarized laser-Compton backscattered photons to assign the E1 or M1 character. Furthermore, we employ inelastic alpha-particle scattering experiments to assign an isospin character to the observed E1 excited levels. Recently, our group has proposed to use high-Q value beta decay of mothers with a low ground state spin to gain an alternative insight in the levels of the PDR. In a first campaign we were able demonstrate that the PDR plays even a role in reactor physics.
Measurements of lifetimes of nuclear states
many-body system made of tens or hundreds of protons and neutrons, is an excellent laboratory with which to test quantum physics. A consistent description of atomic nuclei, from first principles, across the entire periodic table is still unavailable. There exists, however, a large number of successful models which can be employed to describe specific behaviour in nuclei such as collective or non-collective motion. Experimental nuclear physics looks to measure certain key observables with which to test these models with the aim of improving the models which will ultimately lead to a more consistent description of all observed behaviour.
One such key observable is the excitation energy of the quantised excited states of the nucleus. These are typically obtained from measurements of gamma radiation emitted as the excited nucleus decays towards the ground state. Another, perhaps more stringent, test of nuclear models is obtained by measuring the quantum mechanical transition matrix elements which reveal the overlap in the wavefunctions of the nuclear states. The absolute transition matrix elements are obtained through a measurement of the mean lifetime of the nuclear state. A number of techniques have been developed to measure the mean lifetime of a nuclear level and which technique is employed depends on the timescale over which the nuclear level is expected to decay. The timescales typically range from femtoseconds (10-15 s) to milliseconds (10-3 s).
Members of the nuclear physics group at the University of the West of Scotland (UWS) have been leading efforts to measure nuclear lifetimes using a number of different techniques. Gamma radiation emitted from fast-moving atomic nuclei produced in nuclear reactions is subject to the Doppler effect which can be utilised to measure the lifetimes of some of the shortest-lived nuclear states. These experiments are conducted at large international laboratories around the world.
For many nuclear states, it is not possible to measure the mean lifetime using Doppler-based techniques and more direct, electronic techniques are used. These techniques utilise radiation detectors, which have very fast electronic response to the incident radiation, and state-of-the-art electronics to directly measure the time which elapses between the creation and decay of an excited state. Such experiments are conducted both in the detector development laboratory at UWS and at international laboratories across the world.
Neutron-proton interactions
The Gamma Ray Infrastructure for Fundamental Investigations of Nuclei (GRIFFIN) spectrometer is located in the low-energy ISAC-I experimental hall at the TRIUMF facility in Vancouver, Canada. GRIFFIN incorporates 16 Compton-suppressed high-purity germanium Clover gamma-ray detectors with an efficiency of around 10% at 1 MeV. Several ancillary detector systems can be used with GRIFFIN including PACES (Pentagonal Array for Conversion Electron Spectroscopy) and up to 8 lanthanum-bromide [LaBr3(Ce)] detectors. All detector systems are coupled to a triggerless, high-throughput digital data acquisition system. GRIFFIN is uniquely placed to exploit the high-intensity radioactive ion beams provided by the TRIUMF-ISAC facility. The ability to detect very weak gamma-ray transitions at high resolution makes it particularly useful in elucidating the structure of nuclei populated in beta decay. High-energy transitions approaching the beta-decay Q-value may be observed. Both gamma and beta-decay branching ratios can be measured with high precision, providing constraints (for example) on the angular momentum of excited nuclear states. GRIFFIN is ideal for studying ‘superallowed’ (Fermi) beta decays through measurements of ground state to ground state 0+0+ beta transitions, offering insight regarding the Cabibo-Kobayashi-Maskawa (CKM) matrix and the coupling amplitudes between different quark flavours. Members of the UWS Nuclear Physics Research group are leading efforts to explore the structure of heavy actinide nuclei with GRIFFIN, including radium (Z=88) and thorium (Z=90) isotopes, many of which are proposed to exhibit highly collective, reflection-asymmetric octupole shapes near their ground state. The intensity of actinide beams available at the TRIUMF-ISAC facility are among the highest globally. GRIFFIN may be used together with PACES to recover the intensity of highly-converted nuclear de-excitations. Combined with information regarding the lifetimes of nuclear-excited states from the LaBr3(Ce) detectors, a comprehensive picture of the nucleus emerges.
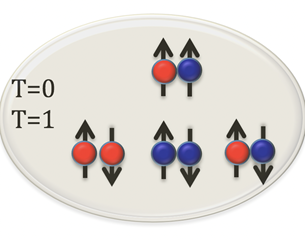
Photo of the east hemisphere of GRIFFIN with BGO Compton-suppression shields installed around the HPGe clovers. Compton-suppressed LaBr3(Ce) detectors are visible to either side of the bottom clover.
Exotic proton-rich nuclei above Sn100
Members of the UWS group have a longstanding interest in the study of the very neutron-deficient nuclei that lie on the nuclear chart just above the doubly magic self-conjugate nucleus 100Sn. The nucleus 100Sn has 50 neutrons and 50 protons, and the nuclei immediately above 100Sn are spherical or weakly deformed nuclei with level structures corresponding to excitations of individual particles in the g7/2, d5/2, and h11/2 orbitals which lie just above the nucleon number of 50. The nuclei close to 100Sn such as 102,103,104Sn exhibit clear single-particle behaviour with irregular excitations with relatively high energies. As more particles are added, collectivity rapidly develops. Already at A=110-112, the nuclei 110Te and 112Te demonstrate a low-lying single-particle like structure but with rotational-like structures at higher energies and higher angular momenta. The addition of several more nucleons leads to rotational structures and deformed ground states in nuclei such as 117,1118,119Cs and 118,119,120Ba. The interplay between the single particle like structures close to 100Sn and the deformed collective rotational structures that develop lead to interesting nuclear structure phenomena. There are a range of compelling physics motivations to study the nuclei in this region. For example, the nuclei with similar numbers of neutrons and protons are expected to demonstrate neutron-proton interactions which can be apparent in a number of ways, for example in the behaviour of aligned angular momenta. Another area of considerable interest is the potential for octupole correlations to develop between particles in the close-lying d5/2 and h11/2 orbitals which have a difference in orbital and total angular momenta of 3 units, and which have opposite parities. Octupole correlations in this region are expected to be maximized at N=Z=56 in the nucleus 112Ba. We are conducting a programme of measurements in these nuclei in experiments with the Jurogam spectrometers at the JYFL Laboratory at the University of Jyväskylä in Finland, with Gammasphere at the Argonne National Laboratory in the USA, and with Galileo at the Legnaro National Laboratory in Italy. In our experiments, we are often exploiting the fact that there is an island of proton and alpha emitters in this region which facilitates the study of these nuclei by the ultra-sensitive technique of recoil-decay tagging. We have recently studied a number of nuclei using these methods such as 112,113Cs and 111Xe. We are planning to continue this programme of research with Jurogam-3 and the new MARA vacuum filled separator at the JYFL Laboratory, and with AGATA at Legnaro.
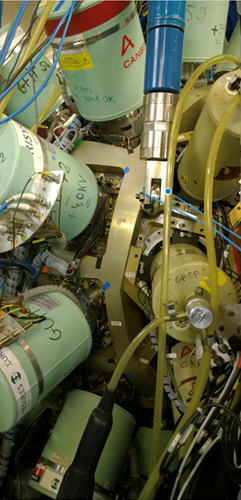
Some of the HPGe detectors of the Jurogam gamma-ray spectrometer at the JYFL Laboratory at the University of Jyvaskyla in Finland.
AGATA – the Advanced Gamma Tracking Array
The UWS group is a member of the AGATA collaboration. AGATA is the Advanced Gamma Tracking Array and is the world’s most powerful and sensitive gamma-ray spectrometers for use in research of the study of nuclear structure and nuclear research. The AGATA collaboration consists of over 40 different institutions in around 12 different countries. The construction of AGATA is a large-scale scientific and engineering endeavour which is being realized in phases over a number of years. When complete, AGATA will consist of 180 high-volume high-purity germanium (HPGe) detectors. Unlike previous gamma-ray spectrometers, AGATA will forego the use of Compton-suppression shields in favour of the use of gamma-ray tracking. Instead of vetoing (throwing away) any gamma rays that are scattered out of an HPGe detector and therefore partially detected, any scattered gamma rays in AGATA are scattered into another HPGe detector, and the gamma ray is tracked and reconstructed. All of the 180 HPGe detectors of AGATA are packed closely together to cover as much of the complete solid angle as possible. The science reach of AGATA is wide-ranging covering nuclear structure, nuclear reactions, and nuclear astrophysics. To further broaden the scope of the science that can be achieved, AGATA is operated in campaigns at different laboratories in Europe. The initial demonstration phase of AGATA too place at the Legnaro National Laboratory in Italy. It has since been operated in campaigns at GSI (Germany) and at GANIL (France) where it is currently operational. In late 2021, AGATA will be moved back to the Legnaro National Laboratory in Italy, where it will be operated at first with stable beams from the Tandem-ALPI accelerators, before being used for experiments with the new SPES radioactive-beam facility. The construction of AGATA is about 50% complete. A UK collaboration consisting of the University of Liverpool, the University of York, Daresbury Laboratory, and UWS was recently awarded an STFC Project Grant to fund the next UK contribution of the AGATA array. The UWS group was one of the most active groups in the previous AGATA campaign at Legnaro, leading two experiments, and we anticipate leading a number of experiments with AGATA in the next Legnaro campaign beginning in 2022.
The AGATA Demonstrato at Legnaro National Laboratory, front and side view.
Nuclear astrophysics
This is a new area of research at UWS led by Dr Nara Singh Bondili. The reactions of interest occur at very high temperatures in astrophysical environments and govern the chemical evolution of the universe. Currently, our research is focused on reactions involving light nuclei, and we will extend this to medium-mass nuclei.
One of our interests is based on experiments relevant to one of the unsolved open problems - the 7Li abundance puzzle which describes a disagreement between predictions and observations by a factor of three. Solving the lithium puzzle is a high priority task in nuclear astrophysics that requires accurate knowledge of the 3He(a,g)7Be reaction rate. We are planning a programme of experiments on this reaction and similar reactions such as 3H(a,g)7Li. Our experiments will be conducted at small-scale facilities such as tandem accelerator in Madrid, Spain through the detection of g rays and also at larger-scale facilities such as the DRAGON facility at TRIUMF (Vancouver) through the counting of 7Be ions.
Our research will also be relevant to neutrinos produced in the sun and has connections to the standard model. Hydrogen is turned into helium in the sun at temperatures ~6000K. Several other fusion reactions follow, producing energy that is required to maintain life on earth. The reactions also produce neutrinos that reach earth, which are detected to study physics beyond the standard model of particle physics. The 3He(a,g)7Be reaction rate is required to calculate the number of neutrinos that can be detected on earth using the standard solar model and compare it with the observations.
Experiments to measure the cross sections for astrophysical reactions in the hot-pp chain involving light nuclei, especially, carbon nuclei are currently being planned through a high-energy beta detection method that is under development.
Image 1: A chamber used for the fusion reaction in Madrid . A 4He beam passing through 3He gas target produces visible light.
Image 2: The DRAGON separator that is used to identify and count 7Be after the fusion reaction at TRIUMF.
Detector development
Throughout the history of nuclear physics, the techniques and apparatus employed to enhance the understanding of the atomic nucleus have found application in diverse fields ranging from medical imaging and border security to the provenance of antiquities. Members of the nuclear physics group at the University of the West of Scotland have been working successfully with external funding agencies to realise the application of some of the detector technology which has been used as part of their fundamental nuclear physics research programmes. In recent years, the Royal Society and Medical Research Scotland have both funded the development of detector technology which could be employed in future medical devices. The Department of Transport has recently funded the construction of a prototype Compton camera system which could be employed at transportation hubs to monitor the movement of radioactive materials.
Research with SCAPA
The Scottish Centre for the Application of Plasma-based Accelerators (SCAPA) is a major research initiative funded by the Scottish Universities Physics Alliance (SUPA) research pool. Based at the University of Strathclyde, SCAPA is a facility dedicated to the development of next-generation particle accelerator technology. Using extremely high powered lasers, SCAPA uses the laser wakefield acceleration (LWFA) method to provide beams of high-energy electrons and protons. These particle beans are being used to solve unique problems within industrial and fundamental physics research programmes.
As a founding member of SUPA, the University of the West of Scotland (UWS) is a stakeholder in the SCAPA facility but members of the nuclear physics group at UWS play a key role in the planning and running of the facility as members of the Management Executive Committee in addition to providing the nuclear physics expertise to the SCAPA staff.
Members of the UWS nuclear physics group are also leading a project to investigate the potential of LWFA in the development of a new modality for the simultaneous treatment and imaging of cancerous tumours within the body. This project will use the SCAPA facility as the primary laboratory in addition to using other accelerators such as CLEAR at CERN.
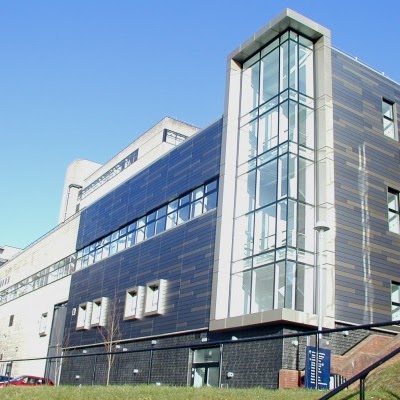
The SCAPA laboratory in the John Anderson Building at the University of Strathclyde in central Glasgow.
RESEARCH PARTNERS
Some of our main collaborators are listed below.
- University of Liverpool (UK)
- University of Manchester (UK)
- University of Surrey (UK)
- University of Brighton (UK)
- University of Strarthclyde (UK)
- University of York (UK)
- Daresbury Laboratory (UK)
- University of Jyvaskyla (Finland)
- University of Padova (Italy)
- TU Darmstadt (Germany)
- Institut Laue Langevin, Grenoble (France)
- Legnaro National Laboratory (Italy)
- Argonne National Laboratory (USA)
- TRIUMF Laboratory (Canada)
- ISOLDE-CERN (Switzerland)
PhD Projects
We welcome applications from PhD students. We have a variety of PhD projects in fundamental and applied nuclear physics, and some of our available projects are described in the School of Computing, Engineering, and Physical Sciences PhD Project Catalogue at https://www.uws.ac.uk/study/school-of-ceps-phd-projects/.
Outreach
We are happy to provide presentations, seminars, events, or visits related to our research on request. For more details please contact John.F.Smith@uws.ac.uk.